Researchers from Google’s Quantum AI team, in collaboration with physicists at Stanford, Princeton, and other universities, announced using Google’s Sycamore quantum computer to demonstrate a genuine ‘time crystal’ for the first time. The researchers revealed the discovery of “time crystals” in a preprint on the arXiv website titled “Observation of Time-Crystalline Eigenstate Order on a Quantum Processor,” which has yet to be peer-reviewed.
A time crystal is an object made of a novel phase of matter that moves in a regular, repeating cycle indefinitely and without consuming any energy. Time crystals are stable, yet the atoms that make them up are continually changing, unlike other phases of matter that are in thermal equilibrium. The study co-author and Max Planck Institute for the Physics of Complex Systems director Roderich Moessner, exclaims that this defies the second law of thermodynamics, which states that systems naturally tend to settle in a state known as “maximum entropy.” This entropy is characterized by randomness and a low useful state.
To put it another way, regular (space) crystals are a collection of molecules or atoms that form a repeating pattern in space. On the other hand, a time crystal is a collection of molecules or atoms that create a regular, repetitive pattern but in ‘time.’ That is, they will sit in one pattern for a while before switching to another and repeating the process. For example, let’s consider an ice cube analogy where an ice cube exists as a solid and melts to become liquid water that can be frozen into ice cubes again. Here the states of water involve a transfer of energy. However, in a time crystal, there will be a change between two configurations back and forth but with no energy usage or energy loss.
Nobel Prize-winning MIT professor Frank Wilczek first proposed time crystals in 2012 as temporal analogs of ordinary space crystals. When realized in a time-independent (energy-conserving) system, time crystals can violate continuous symmetry and discrete-time translation when implemented in a periodically driven (Floquet) system.
According to Quanta Magazine, the time crystal is composed of three main components. First, a row of particles with different magnetic orientations is locked into a combination of low- and high-energy structures. This is referred to as a “many-body localization.” Next is flipping the orientations of those particles that results in creating a mirror version termed as eigenstate order. It’s effectively a secondary many-body localized state. Last but not least, there’s the use of laser light. This enables the states to cycle — from normal to mirrored and back — but without consuming any of the laser’s net energy. The result of this gives us Floquet crystal, which was achieved in 2016. In the said experiment, the researchers then cooled a string of ytterbium ions to their ground state and noticed that the ions could still interact with each other.
Quantum computing has been an asset in performing complex calculations, like drug discovery, climate modeling, financial optimization, or logistics. A couple of years ago, Google’s Sycamore made headlines when Google revealed the quantum computer had achieved quantum advantage (previously known as quantum supremacy). Sycamore could perform calculations in 200 seconds that would take 10,000 years on the fastest supercomputers.
Read More: Microsoft’s Gooseberry Treat For Quantum Computing
What makes quantum computers interesting is instead of the basic manipulation of ones and zeros in conventional binary-based computers, they employ quantum bits or qubits. These qubits also feature a third state known as “superposition,” which can represent a one or a zero simultaneously. Instead of sequentially analyzing a one or a zero, superposition allows two qubits to represent four situations at the same time. Additionally, qubits are capable of entanglement, which is the coupling of two or more qubits. In this case, one qubit’s state cannot be defined separately from the states of the others, allowing for immediate communication.
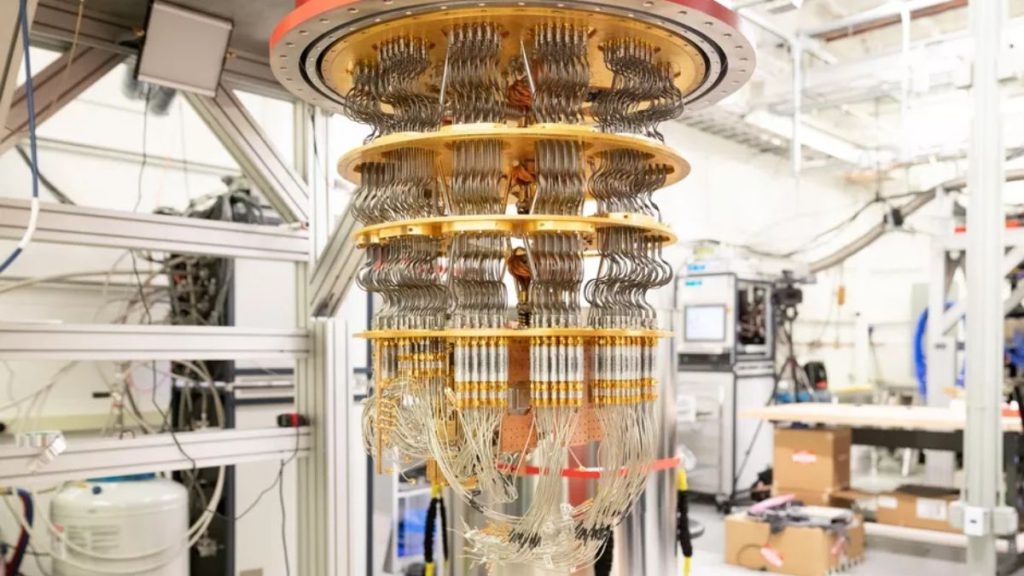
The qubits in Sycamore are superconducting aluminum strips that Google engineers designed to imitate a particle’s spin, which can point up or down, i.e., one or zero. The Google Quantum AI team used a chip with 20 qubits as the time crystal for the experiment. First, the team introduced destructive interference by adjusting the intensity of the interactions between the qubits. The researchers then used microwaves to agitate the system, causing it to flip between two “many-body localized states.” The change in spins did not absorb or dissipate energy from the microwaves, leaving the system’s disorder, or entropy, intact.
According to the researchers, their experiment shows that their technology has the potential to manufacture time crystals. If this discovery is confirmed, it may have massive implications in the realm of quantum computing.
Google is not the first to experiment on the possibility of time crystal-powered quantum computing. On July 5, the Delft University of Technology in the Netherlands announced creating a Floquet time crystal out of the nuclear spins of carbon atoms in a diamond rather than in a quantum processor. In comparison to Google’s Sycamore quantum processor’s time crystal, the Delft system is more restricted and compact.
While the Sycamore feat has caught the attention of the scientific community, experts worry that the decoherence in Google’s processor can result in a decay in the quantum states of the qubits. This implies that time crystal oscillations would ultimately die out when the system is hampered by the environment. However, according to the preprint, this issue might be addressed if the CPU becomes more thoroughly isolated.